Growing organic
Nature 412, 517–520 (2 August 2001)
Imagine how useful a plastic transistor could be. Flexible
displays and cheap, all-plastic smart cards are just a few possibilities.
Last year, the Nobel Prize for chemistry went to Heeger, MacDiarmid
and Shirakawa for their discovery of electrically conducting
polymers, the committee predicting "consequences of great benefit
to mankind".
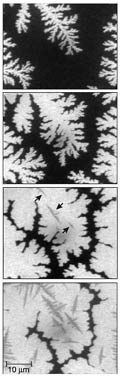 |
Pentacene crystals grow in fractal
formation on a silicon surface. Dark areas appear as the
second layer begins to grow on top of the first layer.
|
But still most microelectronic devices are made of inorganic
semiconducting materials. Plastic transistors are already showing
promise for applications , but their speed of operation is
slow compared to their inorganic counterparts, limiting their
utility. The problem stems from the difficulty in making organic
crystals of high quality in thin-film form. But that may be
about to change: this week in Nature, Frank Meyer zu
Heringdorf and colleagues report progress in understanding organic
thin-film growth through detailed studies of the semiconducting
molecule pentacene (C22H14), a chain-like
aromatic molecule made of five benzene rings.
Using photoelectron emission microscopy, with a field of view
of up to 65 µm and a resolution of 125 nm, the authors watched
the build-up of pentacene on a Si(001) surface over a timescale
of minutes. First, stable two-dimensional islands of pentacene
nucleate on the surface, each island forming a one-layer-thick
pentacene crystal. The islands branch out like fractals, but
before they touch, a second layer nucleates (typically when
the first layer coverage reaches about 60%). Eventually, the
trenches between islands are filled in, leaving grain boundaries
that act as barriers to charge flow — and which would hinder
the electrical performance of transistors made from such films.
Meyer zu Heringdorf et al. noticed a dead-time between
the start of deposition and the nucleation of pentacene islands.
Further imaging revealed that the first-deposited pentacene
molecules are adsorbed at reactive sites (where there are 'dangling
bonds') on the Si(001) surface. Only when all these sites are
neutralized by the adsorption of pentacene can the first layer
of the thin crystalline film start to grow. Treating the Si
surface with cyclohexene or using a different substrate such
as SiO2, which has no dangling bonds, got rid of
the dead-time and produced smoother films.
Also reported in the paper is the successful growth of films
with grain sizes reaching about 0.1 mm — about 100 times larger
than in previous work, and opening up the possibility of fabricating
an organic transistor entirely contained within a single grain.
The authors conclude that their observations will speed up the
development of workable organic thin-film devices.
letters to nature
Growth dynamics of pentacene thin films
The recent demonstration of single-crystal organic optoelectronic
devices has received widespread attention. But practical applications
of such devices require the use of inexpensive organic films
deposited on a wide variety of substrates. Unfortunately, the
physical properties of these organic thin films do not compare
favourably to those of single-crystal materials. Moreover, the
basic physical principles governing organic thin-film growth
and crystallization are not well understood. Here we report
an in situ study of the evolution of pentacene thin films,
utilizing the real-time imaging capabilities of photoelectron
emission microscopy. By a combination of careful substrate preparation
and surface energy control, we succeed in growing thin films
with single-crystal grain sizes approaching 0.1 millimetre (a
factor of 20–100 larger than previously achieved), which are
large enough to fully contain a complete device. We find that
organic thin-film growth closely mimics epitaxial growth of
inorganic materials, and we expect that strategies and concepts
developed for these inorganic systems will provide guidance
for the further development and optimization of molecular thin-film
devices.
FRANK-J. MEYER ZU HERINGDORF, M. C. REUTER & R. M. TROMP
Nature 412, 517-520 (2 August 2001). |